[Updated: February 11, 2014]
Technical Equipment: On-site Measurements
Contents:
- Introduction
- Equipment Maintenance and Calibration
- Direct-Reading Instrumentation for Air Contaminants
- Air Velocity Monitors/Indoor Air Quality (IAQ) Assessment Instrumentation
- Vibration Monitors
- Heat Stress Instrumentation
- Nonionizing Radiation Monitors
- Appendix A Instrument Chart
- Appendix B Chemical Warfare Agent Detection
- Appendix C Biological Agent Detection
- Appendix D Ionizing Radiation Monitors and Meters
I. Introduction
The purpose of this chapter is to provide a broad overview of the types of equipment and instrumentation available for use by OSHA personnel. This information is not a comprehensive resource for specific types of instrumentation, nor is it intended to replace the owner’s manual. Rather, its purpose is to provide a broad understanding of the principle of operation for the particular type of equipment and an understanding of the capabilities and limitations of the equipment. End users should always follow the owner's manual and manufacturer recommendations regarding the specific operation and maintenance of the equipment being used.
The sections which follow discuss various types of instrumentation. Calibration and battery maintenance are discussed in Section II. Section III discusses direct-reading instruments used for assessing chemical and particulate-type air contaminants. Section IV reviews equipment used to support ventilation and indoor air quality (IAQ) assessments. Sections V and VI describe vibration monitors and heat stress monitoring equipment, respectively. Section VII describes instrumentation for detecting nonionizing (e.g., radiofrequency) radiation.
Appendix A provides a useful reference chart which summarizes the various types of instrumentation available and typical applications. Appendices B and C describe specialized tools used to evaluate chemical and biological warfare agents, respectively. Use of these tools is overseen by the Salt Lake Technical Center (SLTC) Health Response Team (HRT) and OSHA’s Specialized Response Teams (SRTs). Appendix D describes equipment used for detection of ionizing radiation, which is also overseen by the HRT.
Note that noise monitoring equipment is NOT discussed in this chapter. Chapter 10 contains the discussion of noise monitoring equipment. Also, this chapter does NOT discuss air sampling methods for collection of samples for off-site laboratory analysis. Active and passive (diffusive) personal air sampling methods for air contaminants are discussed in Chapter 1.
NOTE: Any discussion regarding a specific manufacturer’s product is not meant to imply an endorsement or approval by OSHA, but merely reflects the need to convey specific information which is pertinent to the particular type and brand of instrumentation available for OSHA personnel.
The CTC serves as a source of technical information for instruments and measurement technology. Much of the equipment and instrumentation discussed in this chapter is available from the CTC through the Agency Loan Equipment Program (ALEP), which may be found on the OSHA Intranet.
Hazardous (Classified) Locations
Certain workspaces may contain a flammable or explosive atmosphere due to the accumulation of flammable gases or vapors, or combustible dusts or fibers, and are termed "Hazardous locations." Hazardous locations are classified into Class and division based on the type and severity of the explosion hazard as described in 29 CFR 1910.307. Sparks from ordinary battery-powered portable equipment commonly used by CSHOs, including cameras, cell phones, tablets and laptop computers, may serve as an ignition source, and must never be brought into a hazardous location.
Nationally Recognized Testing Labs test and approve electrical equipment for use in hazardous locations (see 29 CFR 1910.7) Approved equipment, sometimes referred to as intrinsically safe, must be marked with the Class and Division number for which it is approved. Never bring portable monitoring instruments into a hazardous location without first confirming that the instrument is approved for use in that environment. Use only the type of battery specified on the safety approval label, and replace batteries in a nonhazardous area.
II. Equipment Maintenance and Calibration
Service intervals for equipment may be determined by checking the CTC webpage for Equipment Servicing Information. Repairs are generally conducted by the CTC.
Most equipment is calibrated periodically by the CTC, generally on an annual basis. The CTC applies a calibration sticker which includes the due date for the next calibration. Before using field instrumentation, check the calibration sticker and ensure that the instrument is within its calibration due date. Some equipment must be field calibrated or serviced prior to use. Consult the instrument equipment manual to determine what field calibration is needed. If the equipment fails field calibration, consult the CTC for guidance.
Limitations of Batteries
Proper battery maintenance is essential to ensure proper performance of battery-powered equipment during field use. A variety of battery types are used in different types of portable equipment, as specified by the instrument manufacturer. All battery types will self-discharge to some degree during periods of prolonged storage.
Non-rechargeable batteries need to be removed from the instrument prior to prolonged storage in order to prevent battery leakage which could damage the instrument. Many instruments powered by non-rechargeable batteries will perform a battery life check when first powered on (otherwise, check with a voltage meter before full-shift sampling). If low, replace the batteries as appropriate. Never mix types (alkaline, carbon zinc, etc.), capacity, or age, as this can have negative effects on all the batteries.
Rechargeable batteries should generally be left on trickle charge mode for storage. Overcharging, by charging for too long a time period at a high charge rate, can damage the battery. Conversely, discharging below a minimum voltage can also damage the battery. In general, avoid both overnight discharging and overnight charging at a high charge rate. Closely follow the recommendations in the equipment manual. In some cases an outlet controller (timer) can be used to ensure that batteries are charged for a suitable length of time.
Rechargeable batteries are most reliable when used at least every two to three weeks. Nickel-cadmium (Ni-Cd) batteries are prone to developing "memory" problems, in which the battery will not hold a full charge unless it is fully discharged before recharging. Ni-Cd batteries may need to be reconditioned by charging/discharging two to four times. New batteries should be conditioned in accord with the same process. Nickel metal hydride batteries generally are less prone to memory problems than Ni-Cd, and typically offer longer run time, but they do have a higher self-discharge rate. Lithium ion and lithium polymer batteries typically have higher energy density, lower rates of self-discharge, and are not prone to memory effects.
Be sure to consult the user’s manual for proper batter care.
III. Direct-Reading Instrumentation for Air Contaminants
Direct-reading instruments or monitors (also called real-time instruments or monitors) provide information at the time of sampling, thus enabling rapid decision-making. These instruments can often provide the trained and experienced user the capability to determine if site personnel are exposed to airborne concentrations which exceed instantaneous (ceiling or peak) exposure limits for specific hazardous air contaminants. Direct-reading monitors can be useful in identifying oxygen-deficient or oxygen-enriched atmospheres, immediately dangerous to life or health (IDLH) conditions, elevated levels of airborne contaminants, flammable atmospheres, and radioactive hazards. Direct reading instruments are particularly useful for identifying point source contamination or emissions. Periodic monitoring of airborne levels with a real-time monitor is often critical, especially before and during new work activities. Direct-reading instruments are useful for performing screening surveys to determine areas where additional evaluation is warranted. Data obtained from direct-reading monitors can be used to evaluate existing health and/or safety programs and to assure proper selection of personal protective equipment (PPE), engineering controls and work practices.
CSHO Safety
Before bringing monitoring equipment or other electrical devices into an area with the potential for an explosion, always check the Class and Division number marked on the instrument. Use only the type of battery specified on the safety approval label, and replace batteries in a nonhazardous area. Do not assume that an instrument is intrinsically safe. If uncertain, verify by contacting the instrument manufacturer or the CTC.
For atmospheres or work surfaces contaminated with hazardous chemicals, use a plastic bag to cover appropriate parts of equipment to limit contamination. Ensure that the plastic bag is not tightly sealed as this can cause back pressure on the air sampling pump (if equipped). Properly decontaminate all equipment to minimize potential contamination of other persons or objects when sampling is complete. To the extent possible, gross decontamination should be performed onsite.
Before using a direct-reading instrument, review information in the instrument manual regarding the following characteristics:
- Battery life - how long can the instrument run from battery power?
- Datalogging - can the instrument record readings electronically? If so, how is this information retrieved?
- Size and weight - how practical will the instrument be for short-term breathing zone measurements? Will a cart be needed for moving the instrument around?
- Sampling wand - does the unit have a sampling hose and probe to allow for remote sampling, or to allow breathing zone measurements for larger instruments?
- Warm-up time - how long does the unit need to be powered on before it can produce accurate readings?
- Response time (lag time) - how long is the delay between exposing the inlet to a contaminated atmosphere and obtaining an accurate reading?
- Sensitivity - can the analyte be detected, and what is the minimum contaminant concentration that can be reliably measured or detected?
- Specificity - can the instrument discriminate between one contaminant and another?
- Interferences - are there chemicals which, if present, may produce false readings? Are there chemicals, dusts, or other conditions which may damage the sensor?
- Environmental conditions - what is the acceptable temperature and humidity range for use of the instrument? Will temperature and humidity affect the accuracy of the readings? What about altitude? Can the unit be used in a dusty environment without damaging it or requiring factory service? Are there filters available to protect the instrument in these situations?
- Hazardous areas - can the instrument be used in electrical classified areas?
The sections which follow describe the principal types of direct-reading instrumentation. The types discussed include the following:
- Photoionization detectors
- Infrared analyzers
- Gas, oxygen and explosibility (combustible gas) monitors
- Detector tubes
- Mercury analyzers
- Dust/particulate monitors
A. Photoionization detectors
Application and Principle of Operation
Photoionization detectors (PIDs) are used for nonspecific detection of a variety of chemicals, particularly hydrocarbons. PIDs are useful for pinpointing contaminant sources, or for identifying concentration gradients throughout a space, because the readout is proportional to the concentration of contaminant present. However, PIDs cannot positively identify contaminants present in an environment. Where more than one airborne contaminant is present, the instrument may not distinguish one from the other.
PIDs use a high energy ultraviolet (UV) light source to ionize chemicals in an airstream. The charged molecules are collected on a charged surface, which generates a current that is directly proportional to the concentration of the chemical in the air being sampled.
The ionization potential (IP) describes the amount of energy needed to induce ionization in a particular chemical. If the energy of the UV lamp is greater than or equal to the IP of the chemical being sampled, then the chemical will be detected. PIDs may be configured with lamps of different energies. Typical lamp energies are 9.5, 10.6, and 11.7 electron volts (eV). The higher the lamp energy, the greater the number of chemicals that can be detected. For example, benzene (IP 9.25 eV) can be detected with a 9.5 eV lamp, while methylene chloride (IP 11.35 eV) requires use of the 11.7 eV lamp. In general, higher energy lamps have a much shorter lifespan than lower energy lamps. Further, the lamp energy must be lower than background atmospheric gases to be of practical use. For example, the IP of carbon monoxide is 14.01 eV, while the IP of molecular oxygen is 12.08 eV; because the IP of carbon monoxide is higher than for oxygen, a lamp that could ionize carbon monoxide would not be useful for quantifying parts per million (ppm) concentrations of carbon monoxide in the presence of percent concentrations of oxygen (1 percent = 10,000 ppm). Similarly, the IP of many chlorinated hydrocarbons may be too high to be detected by use of a PID.
The amount of electric current (signal response) generated in a PID varies with the chemical to which the PID is exposed, along with the lamp energy. The response factor is the ratio of the detector response for a particular chemical relative to a reference gas, usually isobutylene. The signal response must be multiplied by the response factor to quantify the concentration of the contaminant of interest. Response factors for a large number of chemicals are pre-programmed into the instrument. When sampling in an environment where a single identified gas is known to be present, select the display name for that gas and the readout will be automatically corrected using the response factor for that gas. For chemicals which are not preprogrammed into the instrument, the response factor should be determined by exposing the detector to a known concentration of the gas of interest, by preparing a bag sample (i.e., using a nonreactive Tedlar® bag). Follow the process described in the instrument manual.
Please note that the response factor also depends on the lamp energy. Ensure that the instrument has been set for the energy of the lamp which has been installed. If an incorrect response factor is applied, the displayed reading would significantly under- or over-estimate the concentration of the contaminant in question. The instrument manual includes a table listing the IP and response factor for a variety of common chemicals.
Calibration
PIDs are calibrated using a reference gas, usually isobutylene. Because the response is linear with concentration, a two point calibration is sufficient. Zero gas contains 0 ppm of contaminant, while span gas contains a specified concentration of the reference gas. Use the calibration gases available through the Agency Expendable Supplies Program (AESP) that are specified for the instrument you are using. Calibration gases are typically delivered in nonrefillable 1 liter cylinders. Be sure the gas has not exceeded the expiration date marked on the cylinder. When multiple sensors are present on the same instrument, the right gas mix is necessary to ensure that the span gases for the different sensors do not adversely affect other sensors on the same instrument.
In most cases a calibration check is sufficient, in which the instrument is "zeroed" in fresh air (such as an office environment), then the calibration gas is applied, and if the reading matches the concentration of the span gas, full calibration is unnecessary. Perform a calibration check before each day’s use. If the reading is off, perform a full calibration using zero gas and span gas. Follow the instructions in the manual to enter calibration mode; the unit will auto calibrate, that is, it will internally adjust the signal response so that the displayed reading matches the span gas concentration.
Performing a calibration check using the chemical of interest is recommended where practical, but may not be necessary or possible. In a well-ventilated area, prepare a bag sample of known concentration of the chemical of interest, select that chemical from the instrument library, and ensure that the reading matches the bag concentration.
Special Considerations
Photoionization sensitivity is dependent upon the age of the lamp and cleanliness of the lamp window. Over time, the output of the lamp will be reduced. A spare lamp is sometimes included in the case. Also, the accumulation of organic deposits or buildup of film on the surface of the lamp will reduce sensitivity.
PIDs are also affected by high humidity. For the most sensitive results, it is best to zero the instrument using representative air; that is, zero the instrument in the field in a "clean" area of similar temperature and humidity. The lamp may need to be cleaned more frequently when used in a high temperature, high humidity environment.
Consult the user manual regarding potential interferences. Water vapor, carbon dioxide, methane, and carbon monoxide can all produce a low reading for the gas of interest if present in the air being sampled due to quenching by these non-ionizable gases.
Maintenance
Follow the manufacturer's recommendations for maintaining the detector in optimal condition. This will include routine cleaning of the UV lamp using methanol and frequent replacement of the dust filter. Exercise caution in cleaning the lamp window, as these are fragile. The exterior of the instrument can be wiped clean with a damp cloth and mild detergent, if necessary. Keep the cloth away from the sample inlet and do not attempt to clean the instrument while it is connected to a power source.
B. Infrared Analyzers
Application and Principle of Operation
Infrared (IR) analyzers are useful for measuring a broad range of inorganic and organic chemicals in air. The sensitivity of IR analyzers can be sufficient to quantify chemical concentrations below the OSHA Permissible Exposure Limits (PEL) for many chemicals. IR analyzers can also be used to identify unknown chemicals by using spectral matching. Due to their weight (approximately 10 kg), IR analyzers are most suitable for area sampling rather than personal sampling, although they can be used to analyze a bag sample of contaminated air collected in the breathing zone of a worker (e.g., using a Tedlar® bag). IR analyzers can be used for continuous sampling, although battery life is generally not sufficient for full-shift sampling. Some of the routine applications for IR analyzers include measuring carbon dioxide in IAQ assessments; waste anesthetic gases and vapors including nitrous oxide, halothane, enflurane, penthrane, and isoflurane; and fumigants, including ethylene oxide, ethylene dibromide, chloropicrin, and methyl bromide. IR analyzers are also used in tracer gas studies, such as fume hood performance testing (although these studies are not generally conducted by CSHOs).
IR analyzers operate by passing IR radiation generated from a heated metal source through a gas sample. The IR radiation is absorbed by the chemical at specific wavelengths determined by the type of bonds present in the molecule. The absorbance is proportional to the concentration of the chemical in the sample. The portable IR analyzer is preloaded with a library of known chemicals; to quantify a known chemical in the environment, the user selects the appropriate wavelength for that chemical from the library. The wave number, or number of wavelengths in one centimeter, is commonly used to describe IR spectra. The wave number is the reciprocal of the wavelength and is expressed in cm-1. The infrared spectrum typically used in infrared analysis ranges from the far infrared region at 400 cm-1 (25 micrometers) to the near infrared region 4,000 cm-1 (2.5 micrometers).
The sensitivity (detection limit) can be increased by increasing the path length through which the light source travels. The Miran SapphIRe portable ambient analyzer can measure at a path length of either 0.5 meters or 12.5 meters. Generally the response time is slightly slower for the longer path length.
Where multiple chemicals may be present in the environment, interference can be a problem. A unique absorbance wavelength must be identified to distinguish one chemical from another. The instrument may offer more than one wavelength for measuring concentrations of the same chemical, in order to avoid interferences from different chemicals. The user needs to assess what other chemicals are likely to be present and select the wavelength least likely to have interferences. In some instances, a weaker absorbance band at a different wavelength is chosen to measure a chemical in air, if that alternate wavelength is uniquely absorbed by the chemical of interest.
The selected wavelength for analysis of a chemical is chosen both because the chemical of interest has sufficient absorbance at that wavelength and sufficient specificity to exclude the absorbance of other chemicals. For example, acetone in air absorbs IR at both 8.4 and 11.0 micrometers. If methyl acrylate was also known to be present in the air, the 11.0 micrometer IR wavelength would be selected because methyl acrylate absorbs at 8.4 micrometers.
An IR analyzer can be used to identify unknown chemicals by matching measured spectral absorbance with spectra for known chemicals. The Miran SapphIRe ThermoMatch spectrum correlation software includes 150 common industrial chemicals, and additional spectra can be added by the user. Please note that the ThermoMatch feature is NOT intrinsically safe and must not be used in electrical classified areas.
Calibration
The Miran SapphIRe analyzer is pre-calibrated for a list of chemicals which are stored in the instrument library. A calibration check can be performed using the sampling loop kit. The sampling loop kit recirculates a known volume of air and allows the injection of a known amount of a volatile liquid or gas into the IR sampling cell. Instrument zeroing is performed by using a charcoal filter attachment to remove chemicals from the air. Conduct field calibration in accordance with the manufacturer's recommendations.
Special Considerations
Infrared analyzers may not be specific for the chemical of interest because other chemicals present in the work environment air may also absorb at the same wavelength. Cell window degradation will occur if the analyzer is used in the presence of ammonia and many alkyl amines, such as methyl amine.
Maintenance
Field maintenance is limited to replacement of the zeroing filter after a specified number of uses and replacement of the particulate filter in situations where adsorbed particulates or non-volatile liquids may have contaminated the filter surface.
C. Gas, Oxygen and Explosibility (Combustible Gas) Monitors
A variety of hand-held monitors are supported by the CTC for single, dual, or multi-gas monitoring. Single or dual gas monitors are available from the CTC which can monitor carbon dioxide, carbon monoxide, hydrogen sulfide, and a variety of additional toxic gases. Multi-gas monitors incorporate separate sensors for oxygen, combustible atmosphere, and up to three toxic gases in the same hand-held monitor. The sample concentration is displayed in ppm, percent oxygen or percent LEL (Lower Explosive Limit), as applicable. The monitors are available through the Agency Loan Equipment Program ALEP and the Agency Technical Equipment Procurement Program (ATEPP).
Multi-gas monitors typically feature datalogging capability, as well as audible and/or visual alarms that warn of IDLH or time-weighted average toxic gas concentrations, low oxygen levels, LEL conditions, or malfunction. These monitors may operate in passive (diffusive) mode, or in active mode, in which a pump module draws air across the sensors. Active mode speeds the response time on the meter, but care must be taken to avoid drawing particulates into the monitor. Use active mode for remote sampling of a hazardous atmosphere: introduce the extendable wand, or probe attached to the meter by tubing, into the hazardous atmosphere while the user remains outside the hazardous area (e.g., confined spaces).
Calibration gases for both multi and single gases are available through the AESP. Ensure that the calibration (span) gas is intended for the meter make and model you are using. This is particularly important for multi-gas use because some gases in the mixture can adversely affect other sensors in the same meter. Span Gas Cylinder Recycling is available for empty span (calibration) gas cylinders obtained through the AESP.
Order of testing:Confined spaces, such as sewers and well pits, commonly contain a hazardous atmosphere which may be oxygen deficient and contain a flammable or toxic gas. Many flammable gas sensors are oxygen dependent and will not provide reliable readings in an oxygen deficient atmosphere. Therefore, oxygen content must always be determined before taking combustible gas readings. Flammable gases and vapors are tested second because the risk of fire or explosion is typically more life-threatening than exposure to toxic air contaminants. Monitoring for toxicity is usually conducted last. This monitoring process is greatly simplified by using a multigas monitor containing sensors for oxygen, LEL, and the relevant toxic gases.
The sections which follow describe in further detail the various sensor types which may be installed on a hand-held monitor. Oxygen sensors are discussed first, followed by combustible gas (explosibility) sensors, and lastly, toxic gas sensors.
-
Oxygen Sensors
Application and Principle of Operation
Oxygen sensors are typically based on electrochemical (galvanic) cells. The generated current in the sensor, which is produced from an oxidation reaction, is directly proportional to the rate of oxygen diffusion into the cell. Most meters are calibrated to measure oxygen concentrations between 0 and 25 percent by volume in air. Normal air contains about 20.9 percent oxygen. Meter alarms are usually set to indicate an oxygen deficient atmosphere at concentrations lower than 19.5 percent and an oxygen rich atmosphere at concentrations greater than 23.5 percent. Oxygen concentrations below 19.5 percent may result in difficulty breathing and impaired judgment. Oxygen concentrations below 16 percent result in rapid heartbeat and headache. Sudden physical exertion in an oxygen deficient environment may lead to loss of consciousness. Oxygen concentrations below 12 percent will bring about unconsciousness rapidly and without warning, and are considered IDLH. Oxygen enriched atmospheres present a fire and explosion hazard because ordinary combustible materials will burn more rapidly.
Calibration
Calibration is typically accomplished using fresh outdoor air (20.9 percent oxygen). Calibrate immediately before testing at or near the temperature of the tested atmosphere.
Maintenance
Oxygen sensors are inherently self-consuming and generally last from six to 12 months. When the unit cannot hold calibration, return it to the CTC for sensor replacement and repair (i.e., complete an Instrument Service Request).
-
Explosibility (combustible gas) Sensors
Application and Principle of Operation
Combustible gas sensors use an oxidizing catalyst such as platinum or palladium. Combustible gas meters measure flammable gas concentration as a percentage of the LEL of the calibrated gas. When possible, to maximize the accuracy of the combustible gas readings, calibrate the instrument with the gas that will actually be monitored. If monitoring for combustible atmospheres other than the reference gas (i.e., calibration gas) consult the manufacturer’s instructions and correction charts/curves to determine a more accurate reading of the true gas concentration. Please note that LEL values for most flammable gases and vapors are a few percent in air (i.e., tens of thousands of ppm) and are NOT appropriate for assessing PEL concentrations of flammable toxic gases.
Calibration
Before using the monitor each day, calibrate it with an appropriate calibration gas as described in the user manual or in consultation with CTC. Follow the instructions in the user manual.
Consult the instruction manual before calibration, and ensure that the calibration gas is introduced at the proper pressure and flow rate, using the appropriate regulator and adaptors. Overpressurization can damage the sensor. For some instruments with an active sampling pump, the pump must be disconnected from the sensor and the span gas flow rate set to match the sampling rate of the pump.
Special Considerations
- Silicone compound vapors and sulfur compounds will cause desensitization of the combustible gas sensor and produce erroneous (low) reading.
- High relative humidity (90 percent to 100 percent) causes hydroxylation, which reduces sensitivity and causes erratic behavior including inability to calibrate.
- Oxygen deficiency or enrichment, such as in steam or inert atmospheres, will cause erroneous readings for combustible gases. Always note the oxygen concentration reading before assessing the LEL reading.
- In extraordinary circumstances, gas concentrations above the Upper Explosive Limit (UEL) may give a false reading indicating a noncombustible atmosphere; be aware that if air is suddenly introduced into such a space, the atmosphere can quickly become explosive.
- Vapors from liquids with flash points above 90°F such as turpentine, diesel fuel, and jet fuel, may not be adequately detected by combustible gas sensors. Use of a photoionization detector may be more appropriate.
- In drying ovens or unusually hot locations, solvent vapors with high boiling points may condense in the sampling lines and produce erroneous (low) readings. Consider taking readings at several different locations around the oven.
- High concentrations of chlorinated hydrocarbons, such as trichloroethylene or acid gases such as sulfur dioxide, will depress the meter reading in the presence of a high concentration of combustible gas.
- High molecular weight alcohols can burn out the meter's filaments.
Maintenance
The instrument requires no short-term maintenance other than regular calibration and recharging of batteries. Use a soft cloth to wipe dirt, oil, moisture, or foreign material from the instrument.
-
Toxic Gas Sensors
Application and Principle of Operation
Available toxic gas sensors include sensors for carbon monoxide, hydrogen sulfide, nitrogen dioxide, sulfur dioxide, chlorine, chlorine dioxide, phosphine, ammonia, hydrogen cyanide and hydrogen. While the toxic gas sensors are interchangeable, these instruments are not easily serviced in the field. Should a different gas sensor need to be installed, return the instrument to the CTC for a change of sensors.
Toxic gas sensors generally use an electrochemical (voltammetric) sensor or polarographic cell to provide continuous analyses. In operation, sample gas is absorbed on an electrocatalytic sensing electrode after passing through a diffusion medium. An electrochemical reaction generates an electric current directly proportional to the gas concentration.
Interference from other gases can be a problem. Before use, consult the user manual to identify interfering chemicals of concern. Some interfering compounds can result in false positive readings. In other cases, the sensor can be damaged, or "poisoned" by exposure to certain compounds, in which case it will need to be returned to the CTC for sensor replacement.
Calibration
Calibration against a known standard is required. Tests have shown the method to be linear; thus, calibration at a single concentration, along with checking the zero point, is sufficient. Calibrate with the appropriate calibration (span) gases before and after each use in accord with the manufacturer's instructions.
The monitor should be calibrated at the altitude at which it will be used. Changes in total atmospheric pressure caused by changes in altitude will influence the instrument's response. The unit's instruction manual provides additional details on the calibration of sensors.
Consult the instruction manual before calibration, and ensure that the calibration gas is introduced at the proper pressure and flow rate, using the appropriate regulator and adaptors.
Overpressurization can damage the sensor. For some instruments with an active sampling pump, the pump must be disconnected from the sensor and the span gas flow rate set to match the sampling rate of the pump.
D. Detector Tubes
Application and Principle of Operation
There is a wide variety of commercially available detector tubes which can be used to measure over 200 organic and inorganic gases and vapors in air. Detector tubes are sealed glass tubes filled with a granular material that is coated with an appropriate indicator chemical that will react with a particular gas or vapor to give a color change. Their operation consists of using a portable pump to draw a known volume of air through a detector tube designed to measure the concentration of the substance of interest. The color change is read in terms of either the length of stain generated inside the tube or the degree of color change. This color change is compared either to a scale printed on the tube or to a reference chart included with the tube kit to determine the airborne concentration.
The pumps can be hand-operated (weight: 8-11 ounces), or they can be an automatic type (weight: about 4 pounds [lbs]) that samples using a preset number of pump strokes. Detector tubes of a given brand are to be used only with a pump of the same brand. A brand of tubes is calibrated specifically for the same brand of pump and may give erroneous results if used with a pump of another brand.
Important considerations for use of detector tubes include measurement accuracy, limits of detection, interferences, temperature and humidity, shelf life, and the time period for which the color stain is stable after the sample is drawn. Always consult the manufacturer’s printed instructions to determine these specifications, along with the required number of pump strokes and the time between pump strokes.
Detector tubes are most useful for screening purposes to determine whether levels of contaminant present in an area warrant further sampling. Detector tubes can also be used for compliance sampling relative to TWA (8-hour time-weighted average), STEL (short-term exposure limit) and Ceiling limits. For example, the SLTC has documented procedures for use of direct-reading devices, including detector tubes, for the measurement and tracking of methylene chloride exposure, which can be found on the OSHA Intranet. This document discusses the specific detector tubes recommended to determine workplace methylene chloride concentrations based on laboratory studies and includes an example calculation of a methylene chloride TWA exposure. The minimum number of pump strokes required to get a positive response near the STEL is also discussed.
Detector tubes are obtained through the CTC AESP. OSHA’s Chemical Sampling Information (CSI) files list specific manufacturer’s models of detector tubes for individual gases/vapors. The specific tubes listed are designed to cover a concentration range near the PEL. Concentration ranges are tube-dependent and can be anywhere from one-hundredth ppm to several thousand ppm. The limits of detection depend on the particular detector tube. Detector tube accuracy varies with tube manufacturer and with each detector tube range.
Before use, refer to the manufacturer’s instructions for the particular tube type. Determine the measurement precision, which is typically +/- 25-35%, and be sure to record the measurement accuracy when recording the sample result on the Form OSHA-93, Direct Reading Report Form. Also, perform a pump leak test as shown in Figure 2.
To use, break the ends with a tube opener, which is generally part of the manufacturer’s sampling kit. Attach the tube to the pump of the correct brand. Tubes generally have a directional arrow printed on each tube. As shown in Figure 1, ensure that the directional arrow is oriented toward the pump. Use the number of pump strokes specified by the manufacturer for that tube type.
On each day of use, before taking measurements, perform a pump leakage test as per the user instruction manual. The general procedure is to insert an unopened detector tube into the pump and attempt to draw in 100 milliliters (mL) of air. After a few minutes, check for pump leakage by examining the pump compression for bellows-type pumps, or return to resting position for piston-type pumps. Automatic pumps should be tested according to the manufacturer's instructions. The leak test procedure is shown in Figure 2.
In the event of pump leakage that cannot be repaired in the field, send the pump to the CTC for repair. Record that the leakage test was performed on the Direct Reading Report Form (OSHA-93).
The Dräger Chip Measurement System is an accurate and reliable hand-held reader based on colorimetric detection which is very useful for spot gas measurements. It combines an electronic-based analyzer with substance specific chips which are available through the AESP. Each chip contains 10 capillaries filled with a reagent system. The results of up to 50 measurements can be stored in a data recorder integrated in the analyzer.
Interferences
A limitation of many detector tubes is the lack of specificity of the chemical indicator. Many indicators are not highly selective and can cross-react with other gases and vapors. Manufacturer’s manuals describe the effects of interfering contaminants.
Figure 1. Proper Insertion of Detector Tube into Pump.
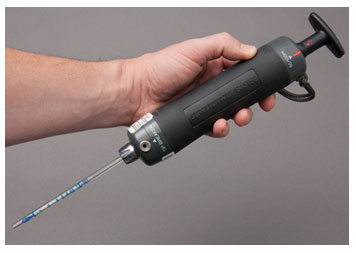
Figure 2. Pump Leak Test.
Temperature and Humidity
Temperature, humidity and pressure can also affect detector tube readings. Read and follow the manufacturer’s instructions regarding corrections that must be made to sample readings for these factors.
Long-Term Sampling
Most detector tubes only give near-instantaneous measurements, and thus will not reflect time-weighted average (TWA) levels of the hazardous substances present. Some long duration tubes for TWA measurements are available. Some are a diffusive/dosimeter type which requires no pump. Others are used with a portable lightweight pump which continuously draws a measured volume of air through the tube. These tubes can be worn by the worker in a special holder. At the end of the shift, the tube can be evaluated to give a TWA exposure for the working day (e.g., see the SLTC procedure, found on the OSHA Intranet, to determine methylene chloride in workplace air by long-term detector tubes).
Another technique which could be used for long-term measurements with detector tubes is to wear a gas sampling bag such as a Tedlar® or Teflon® bag connected to a low flow pump and then periodically measure the concentration of contaminant in the bag (with a detector tube) to get a TWA exposure over the time period worn (e.g., on the OSHA Intranet see the SLTC utilization of direct-reading devices for the measurement and tracking of methylene chloride exposure). The sampling bag is connected to the outlet from a portable sampling pump set to a calibrated flow rate such as 0.05 liters per minute (50 cubic centimeters per minute). Once the long-term bag sample is collected, an air sample could be extracted in the field by connecting the bag to a detector tube and hand pump. Massage the bag to ensure good air mixing before extracting the air sample. This technique can only be used for assessing contaminants that would not react over time inside the bag. Consult the sampling pump manual to ensure that atmospheres that may be damaging to the pump are not drawn into it.
Storage and Shelf Life
Detector tubes normally have a shelf life of one to two years when stored at 25°C. Expiration dates are generally printed on the box or on each tube. In general, avoid excessively low (less than 35°F) or high (greater than 78°F) temperatures and direct sunlight which can adversely affect the properties of the tubes. Refrigerated storage prolongs shelf life. Detector tubes should not be used when they are cold. They should be kept at room temperature for about one hour prior to use. Outdated detector tubes (i.e., beyond the printed expiration date) should not be used.
Calibration
Annually or after any repair or maintenance work, send detector tube pumps to the CTC for calibration (volume verification). Calibration in the field is no longer performed. Consult the CTC if there is reason to suspect that a pump may not be operating properly prior to its scheduled calibration due date.
E. Mercury Analyzers
Application and Principle of Operation
Handheld mercury analyzers can be used for compliance sampling and for source and leak detection. These instruments measure airborne mercury vapor by drawing an air sample over a gold film. The mercury adsorbed onto the gold surface changes the resistance of current flow. The change in resistance is a function of the mass of mercury collected on the gold film. The results can be displayed in milligrams of mercury per cubic meter of air (mg/m3) or total mass of mercury in the air sample collected. Models available through ALEP include the Jerome Model 431X, which has a lower limit of detection of 0.003 mg/m3 and achieves +/- 5 percent accuracy at 0.1 mg/m3; and the Jerome 405-0007, which has a lower limit of detection of 0.5 micrograms per cubic meter of air (μg/m3) and achieves +/- 5 percent accuracy at 25 μg/m3 and +/- 10 percent accuracy at 1 μg/m3.
Potential interferences which can produce a positive reading include chlorine, nitrogen dioxide, hydrogen sulfide, high concentrations of ammonia, and most mercaptans. Depending on the model, various filters are available from the manufacturer to remove chlorine, ammonia, and other interfering contaminants upstream of the sensor.
Calibration
Factory calibration is required annually. Because of the gold film/mercury interaction, the instrument should produce stable, accurate readings without the need for frequent recalibration. A calibration check can be performed using the manufacturer’s Functional Test Kit.
Special Considerations
The instrument must be zeroed before use at the temperature at which it will be used. Both models have a zero air filter which removes mercury vapor, mercaptans, and hydrogen sulfide.
The instrument should be regenerated before and after use because the gold film sensor becomes saturated with mercury. The meter must be placed on line power and the gold film sensor regenerated at elevated temperature. After regeneration, wait half an hour before taking readings to allow the sensor to equilibrate to room temperature.
Maintenance
Routine maintenance includes periodic replacement of filters and regeneration of the gold film sensor to remove mercury after use of the instrument.
F. Dust / Particulate Monitors
-
Aerosol Photometers
Application and Principle of Operation
Aerosol photometers operate by detecting scattered light or infrared radiation. The amount of light reaching the detector is proportional to the number of particles passing through the detection chamber. Also known as nephelometers, these instruments are used to monitor particulate matter such as dusts, smokes, mists, and fumes. Some models can be used for monitoring the respirable fraction of dust, and some are small enough to use for personal exposure monitoring. Results are reported in μg/m3 or mg/m3. Some models are also a fibrous aerosol monitor (FAM), which report the number of fibers per volume of air (e.g., these instruments are useful for real-time measurements of airborne asbestos).
Calibration
Annual factory calibration is arranged by the CTC. Field zeroing prior to use is also required.
Special Considerations
Certain instruments have been designed to satisfy the requirements for intrinsically safe operation in methane-air mixtures.
Relative humidity conditions above 80 percent may result in readings which are higher than the actual dust concentration. Follow the manual for any necessary adjustments.
Maintenance
When the photodetector is not being operated, it should be placed in its plastic bag, which should then be closed. This will minimize particle contamination of the inner surfaces of the sensing chamber.
The unit will be cleaned as part of the annual calibration service. Follow the user manual in regards to field servicing. Excessive buildup of particles in the sensing chamber may affect the accuracy of measurements.
After prolonged operation or exposure to particulate-laden air, the interior walls and the two glass windows of the sensing chamber may become contaminated with particles. Although repeated updating of the zero reference following the manufacturer’s procedure will correct errors resulting from such particle accumulations, this contamination could affect the accuracy of the measurements as a result of excessive spurious scattering and significant attenuation of the radiation passing through the glass windows of the sensing chamber.
-
Condensation Nuclei Counters
Application and Principle of Operation
Condensation nuclei counters take particles too small to be easily detected, enlarge them to a detectable size, and then count them. Common uses for this technology include quantitative respirator fit-testing and testing the removal efficiency of high efficiency particulate air (HEPA) filters.
Submicrometer particles are grown with alcohol or another liquid vapor as they pass through a heated saturator lined with alcohol-soaked felt. The alcohol vapor becomes super-saturated and condenses on the particles in a cooled condenser. Optics focus laser light into a sensing volume. As the droplets pass through the sensing volume, the particles scatter the light. The light is directed onto a photodiode which generates an electrical pulse from each droplet. The concentration of particles is counted by determining the number of pulses generated. This instrument is sensitive to particles as small as 0.02 micrometers. However, it is non-specific to variations in size, shape, composition, and refractive index.
A counter totals individual airborne particles from sources such as smoke, dust, and exhaust fumes. Common models operate in one of three possible modes, each with a particular application. In the "count" mode, the counter measures the concentration of these airborne particles. In the "test" (or fit- test) mode, measurements are taken inside and outside a respirator and a fit factor is calculated. In the "sequential" mode, the instrument measures the concentration on either side of a filter and calculates filter penetration.
Calibration
Check the counter before and after each use in accordance with the manufacturer's instructions. This procedure usually involves checking the zero of the instrument. Annual calibration is handled through the CTC.
Maintenance
Reagent-grade isopropyl alcohol for use in these types of instruments can be obtained from the CTC AESP. Isopropyl alcohol must be added to the unit after five to six hours of operation under normal conditions. Take care not to overfill the unit.
A fully charged battery pack will normally last for about five hours of operation. Low battery packs should be charged for at least six hours. Battery packs should not be stored in a discharged condition.
To prepare the unit for long-term storage, follow the instructions in the equipment manual. It may be necessary to dry the saturator felt by installing a freshly charged battery pack without adding alcohol. Allow the instrument to run until the LO message (low battery) or the E-E message (low particle count) appears. Some instruments allow you to remove the alcohol cartridge for storage purposes. Remove the battery pack and install the tube plugs into the ends of the twin-tube assembly.
IV. Air Velocity Monitors/Indoor Air Quality (IAQ) Assessment Instrumentation
NOTE: Always record the barometric pressure and air temperature when using air velocity meters. Refer to the user manual to determine the operating range for temperature and pressure. The measurement uncertainty may be greater when used at temperatures above or below room temperature.
A. Flow Hoods
Application and Principle of Operation
A flow hood or balometer is an instrument used to measure volumetric air flow from supply or exhaust diffusers and grilles. The benefit of using a flow hood is that accurate measurements with a high degree of precision can be quickly obtained without the necessity of measuring grille sizes and conducting repeated velometer measurements over the face of the diffuser or grille. With the flow hood, the user can measure air volume, check HVAC system balance, verify air flow distribution within and between rooms, and in combination with other data, estimate the percent of outdoor air being supplied to a space. Additionally, if the diffuser area is known or measured, an accurate average linear air velocity can be calculated. Such applications may be important in assessing ventilation controls or conducting IAQ investigations. Other useful applications include determining volumetric airflow for dilution ventilation and evaluating airflow patterns to ensure that contaminants are not being pulled into unintended work areas. For more guidance on the appropriate use of flow hoods, please contact the SLTC.
Calibration
No field calibration is available; CTC handles calibration. Periodic factory calibration or equivalent by a laboratory is essential.
Maintenance
These instruments typically require little field maintenance other than battery pack servicing and zero balancing of the analog scales. Check the manufacturer's user manual for details.
B. Thermoanemometers
Application and Principle of Operation
A thermoanemometer (hotwire anemometer) is a handheld device with an extendable wand probe used to measure air speed (velocity). Due to their use of a heated wire, they are generally NOT suitable for use in hazardous areas where intrinsically safe equipment is required. Thermoanemometers can be used to monitor the effectiveness of ventilation systems and local exhaust systems. In general, thermoanemometers are appropriate for measuring laminar (nonturbulent) airflow. Multiple readings must be taken at different points in a plane which is perpendicular to the direction of airflow, and then averaged together. They are valuable when evaluating laboratory hoods for adequate face velocity. When the area of the hood face or exhaust diffuser is known (or measured) the volumetric airflow can be estimated by taking multi-point traverse measurements across the face of the hood or diffuser. A thermoanemometer can also be used effectively to assess downdraft/sidedraft tables and slot ventilation, because these all operate by establishing a stable capture velocity in a defined capture plane (e.g., at a specified distance from the slots). They are not useful for assessing snorkel-type local exhaust, due to generally turbulent airflow in the capture zone of a snorkel. Additionally, if a duct has access ports, the interior duct speed can be estimated by taking a multipoint traverse. To ensure accurate readings, a duct traverse should not be taken within three duct diameters of any elbows, branches, fans or transitions due to turbulent airflow in these locations. For more guidance on the appropriate use of thermoanemometers, please contact the SLTC.
"Ventilation smoke" is available through the AESP and is a helpful complement to the thermoanemometer. Smoke released inside an enclosing hood, such as a laboratory fume hood or spray booth, or outside an exterior hood, such as a snorkel, slot ventilation, canopy hood, downdraft table, or side draft hood, will help visualize whether contaminants will be effectively contained and/or captured. Ventilation smoke will also help determine whether supply air turbulence near a hood may compromise the hood’s effectiveness.
Calibration
No field calibration is available. Equipment should be sent to the CTC for calibration and document retention. The CTC calibration interval is once every two years.
Special Considerations
While thermal anemometers can be very accurate, their accuracy may be adversely affected by air turbulence, temperature variations, or dirty probes. Note the manufacturer’s limitations of use. Thermal anemometers are generally not intended for use in gas mixtures other than air, corrosive atmospheres, or other hazardous gas streams.
Maintenance
These instruments typically require little field maintenance other than battery pack maintenance and zero balancing of analog scales, if applicable. Check the manufacturer's user manual for details.
C. Other Air Velocity Meters
Other types of air velocity meters include rotating vane and swinging vane velometers. These are used infrequently, but may be useful in circumstances where a thermoanemometer cannot be used, for example, corrosive atmospheres or hazardous environments. Also, rotating vane anemometers can be used to assess snorkel-type local exhaust ventilation.
D. Bioaerosol Monitors
Application and Principle of Operation
Assessment of bioaerosols may be useful in certain IAQ investigations. A bioaerosol monitor, usually a two-stage sampler, is also a multi-orifice cascade impactor. This unit is used when size distribution is not required and only respirable-nonrespirable segregation or total counts are needed. Ninety-five to 100 percent of viable particles above 0.8 microns in an aerosol can be collected on a variety of bacteriological agar. Trypticase soy agar is normally used to collect bacteria, and malt extract agar is normally used to collect fungi. Blood agar is typically used for collection of Stachybotrys chartarum. Bioaerosol monitors can be used in assessing sick-building syndrome, or buildings which may have source exposures to molds and bacteria which may be exacerbating or causing illness to the occupants. These samplers are also capable of collecting virus particles. However, there is no convenient or practical method for cultivation and enumeration of viral particles.
The SLTC does NOT perform analysis of biological samples in-house. The SLTC coordinates analysis of biological samples by contracted laboratories. When considering biological sampling, contact the SLTC to discuss sampling requirements, technical support, assessment, and analytical coordination. The SLTC staff will review the sampling and analysis plans with the CSHOs and make recommendations where appropriate. The SLTC offers contracting services for analysis of fungi, bacteria (such as Legionella), and endotoxin. Other services can be arranged on a case-by-case basis.
Calibration
Bioaerosol meters must be flow-calibrated before use, using the same type of sampling media in the sampling train as will be used in the field. This can be done using an electronic calibration system with a high-flow cell.
Special Considerations
Prior to sampling, work with the SLTC HRT to determine the type of collection media required, sampling flow rates and times, and the analytical laboratory that will provide analysis. This specialized equipment is available from the HRT with instructions.
Maintenance
The sampler should be decontaminated prior to use by sterilization, or chemical decontamination with isopropanol.
V. Vibration Monitors
The following sections contain a brief discussion of various types of measurements that are of concern when measuring vibration. Human response to vibration is dependent on several factors including the frequency, amplitude, direction, point of application, time of exposure, clothing and equipment, body size, body posture, body tension, and composition. A complete assessment of exposure to vibration requires the measurement of acceleration in well-defined directions, frequencies and duration of exposure. The vibration will generally be measured along three (x, y and z) axes.
A typical vibration measurement system includes a device (accelerometer) to sense the vibration, a recorder, a frequency analyzer, a frequency-weighting network, and a display such as a meter, printer or recorder. The accelerometer produces an electrical signal in response to the vibration. The size of this signal is proportional to the acceleration applied to it. The frequency analyzer determines the distribution of acceleration in different frequency bands. The frequency-weighting network mimics the human sensitivity to vibration at different frequencies. The use of weighting networks gives a single number as a measure of vibration exposure (i.e., units of vibration) and is expressed in meters per second squared (m/s2).
A. Hand-Arm Vibration
Application and Principle of Operation
Hand-arm vibration will generally be measured when using a hand-held power tool. First, one must determine the type of vibration that will be encountered because a different accelerometer will be used depending on whether an impact (e.g., jackhammer or chipper) or non-impact (e.g., chain saws or grinders) tool is being used. The accelerometer will be attached to the tool (or held in contact with the tool by the user) so the axes are measured while the worker grasps the tool handle. The z axis is generally from the wrist to the middle knuckle, the x axis is from the top of the hand down through the bottom of the hand and wrapped fingers, and the y axis runs from right to left across the knuckles of the hand. The measurement should be made as close as possible to the point where the vibration enters the hand.
The frequency-weighting network for hand-arm vibration is given in the International Organization for Standardization (ISO) standard ISO 5349-1 (Mechanical Vibration - Measurement and Evaluation of Human Exposure to Hand-Transmitted Vibration – Part 1: General Requirements). The human hand does not appear to be equally sensitive to vibration energy at all frequencies. The sensitivity appears to be the highest around 8-16 Hz (Hertz or cycles per second), so the weighting networks will generally emphasize this range. Vibration amplitudes, whether measured as frequency-weighted or frequency-independent acceleration levels (m/sec2), are generally used to describe vibration stress (American National Standards Institute, American Conference of Governmental Industrial Hygienists, ISO, and the British Standards Institution). These numbers can generally be read directly from the human vibration meter used. The recommendations of most advisory bodies are based on an exposure level likely to cause the first signs of Stage II Hand-Arm Vibration Syndrome (white finger) in workers.
OSHA does not have standards concerning vibration exposure. The American Conference of Governmental Industrial Hygienists (ACGIH) has developed Threshold Limit Values (TLVs) for vibration exposure to hand-held tools. The exposure limits are given as frequency-weighted acceleration. The frequency weighting is based on a scheme recommended in ISO 5349-1. Vibration-measuring instruments have a frequency-weighting network as an option. The networks list acceleration levels and exposure durations to which, ACGIH has determined, most workers can be exposed repeatedly without severe damage to the fingers. The ACGIH advises that these values be applied in conjunction with other protective measures, including vibration control.
B. Whole-Body Vibration
Application and Principle of Operation
The measurement of whole-body vibration is important when measuring vibration from large pieces of machinery which are operated in a seated, standing, or reclined posture. Whole-body vibration is measured across three (x, y and z) axes. The orientation of each axis is as follows: z is from head to toe, x is from front to back and y is from shoulder to shoulder. The accelerometer must be placed at the point where the body comes in contact with the vibrating surface, generally on the seat or against the back of the operator.
The measurement device is generally an accelerometer mounted in a hard rubber disc. This disc is placed in the seat between the operator and the machinery. Care should be taken to ensure that the weight of the disc does not exceed more than about 10 percent of the weight of the person being measured.
Calibration
Vibration equipment will not generally be calibrated by the user. These devices will generally be sent back to the manufacturer for calibration on an annual basis.
Special Considerations
The most widely used document on whole-body vibration is ISO 2631-1 (Evaluation of Human Exposure to Whole-Body Vibration – Part 1: General Requirements). These exposure guidelines have been adopted as ACGIH TLVs.
The ISO standard suggests three different types of exposure limits for whole body vibration, of which only the third is generally used occupationally and is the basis for the ACGIH TLVs:
- The reduced-comfort boundary is for the comfort of passengers in airplanes, boats, and trains. Exceeding these exposure limits makes it difficult for passengers to eat, read or write when traveling.
- The fatigue-decreased proficiency boundary is a limit for time-dependent effects that impair performance. For example, fatigue impairs performance in flying, driving and operating heavy vehicles.
- The exposure limit is used to assess the maximum exposure allowed for whole-body vibration. There are two separate tables for exposures. One table is for longitudinal (foot to head; z axis) exposures, with the lowest exposure limit at 4 to 8 Hz based on human body sensitivity. The second table is for transverse (back to chest and side to side; x and y axes) exposures, with the lowest exposure limit at 1 to 2 Hz based on human body sensitivity. A separate set of "severe discomfort boundaries" is given for 8-hour, 2-hour and 30-minute exposures to whole-body vibration in the 0.1–0.63 Hz range.
The ACGIH recommendations are based on exposure levels that should be safe for repeated exposure, with minimal risk of adverse effects (including pain) to the back and the ability to operate a land-based vehicle.
Some general considerations for using vibration equipment include:
- Batteries should always be checked prior to use.
- Be careful with electrode cables. Never kink, stretch, pinch or otherwise damage the cables.
- Remove the batteries from any meter that will be stored for more than a few days.
- Protect meters from extreme heat and humidity.
HRT Availability
The HRT maintains the following vibration analysis equipment:
Larson Davis Human Vibration Meter - HVM100
The Larson Davis HVM is a portable multipurpose meter which can be used for measurement of whole-body vibration, hand-arm vibration, hand-tool vibration, vibration severity and product compliance testing. It will collect and analyze data in accord with the most current ISO requirements for hand-arm vibration and whole-body vibration exposures. It measures three input channels simultaneously, and a fourth channel calculates and stores vector sum information. Single and triaxial accelerometers attach to specialized mechanical mounting adaptors to allow measurement on a wide variety of tools and surfaces.
C. Mechanical Force Gauge for Ergonomic Evaluations
Application and Principle of Operation
Mechanical force gauges are frequently used for a wide range of force testing applications including testing of compressive and/or tensile forces. The gauges may be mounted to a test stand for even greater control and consistent results in repetitive testing applications. An easy to read concentric dial measures clockwise direction only. The dial rotates 360-degrees for tarring. A peak hold button captures peak readings. Usually the gauges are available in pound (lb), kilogram (kg) or Newton units of measure.
Calibration
Gauge accuracy should be checked periodically to ensure that the gauge is within its calibration limits. The calibration can be verified by applying known weight (adjusted for local gravity) to the extension hook. If adjustment is required, the gauge should be returned to the manufacturer for calibration.
VI. Heat Stress Instrumentation
The following sections contain a brief discussion of various types of instruments that may be used for heat and heat stress monitoring. Refer to the OSHA Technical Manual, Section III: Chapter 4 - Heat Stress for additional information on heat-related injuries and illnesses.
Application and Principle of Operation
There are two types of heat stress monitors available through the CTC. One type is a real-time area monitor that measures environmental conditions that contribute to heat stress, and the other is a real-time personal monitor that measures the wearer’s body temperature and/or heart rate. The area monitors (QUEST Model QUESTemp™ 15 or 3M WIBGET™ Model RSS-214) measure indoor and outdoor wet bulb globe temperatures (WBGT). The temperature values can be data logged and the monitor can also be configured to sound an alarm when a predetermined WBGT is reached. The personal monitors provide real-time information on the wearer's physiological condition and work effort by either inserting a probe into the wearer's ear canal to monitor body temperature (QUEST Technologies QUESTemp II™), or wearing a sensor belt around the waist that monitors heart rate and temperature (Metrosonics® hs-383 Personal Heat Stress Monitor). Both personal devices can be programmed to alarm when a predetermined temperature or heart rate is exceeded.
The area monitors work by taking measurements of the ambient temperature, the wet bulb temperature, and the globe temperature, and then using a formula to determine the WBGT. The wet bulb temperature takes into account the effects of humidity on the body's cooling mechanism and the globe temperature accounts for radiant heat on the worker. Outdoors, a WBGT is calculated by multiplying the wet bulb temperature by 0.7, the globe temperature by 0.2, and the dry bulb temperature by 0.1. Because radiant heat from the sun is not a factor indoors (or outdoors without a radiant heat load), the WBGT is calculated differently for indoor environments: the wet bulb multiplier stays the same, the globe temperature is multiplied by 0.3, and the dry bulb temperature drops out of the formula.
Another personal heat stress monitoring system is available through the HRT. The CorTemp™ personal heat stress monitoring system uses an ingestible temperature sensor that is swallowed by the person being monitored. The capsule transmits the worker's core temperature to a receiver on his/her belt, which also receives a heart rate monitor's reading, and then transmits both signals to a receiver monitored by an observer up to 100 yards away. Because the sensor must be swallowed, the CorTemp™ system is considered a medical device and must be used under the supervision of a physician.
Calibration
Most calibration is done annually by the manufacturer. Certain instruments have simple user calibrations that must be performed before each use. The QUESTemp™ 15 is provided with a calibration module that plugs into the monitor. If the temperatures reported by the module and the monitor differ by more than 0.5°C (32.9ºF), the monitor needs to be returned to the manufacturer for calibration. The QUESTemp™ II personal heat stress monitor is calibrated to the user's body temperature every time it is used or if the ambient temperature changes by 10°C (50ºF).
VII. Nonionizing Radiation Monitors
Survey Meters and Personal Monitors
Application and Principle of Operation
Radio Frequency (RF) survey meters are used to measure both electric and magnetic fields from RF sources. RF meters must be selected based on the frequency of the radiation that is to be measured. Meters typically have interchangeable probes for measuring electric and magnetic fields. Some meters and probes are capable of performing spatial and temporal averaging for multiple frequencies and displaying measurement results in percent of exposure from guidelines recommended by one of several consensus standards.
RF personal monitors are used to measure personal RF exposures. These monitors are worn on the belt and continuously log personal exposures and provide an exposure result using a shaped frequency response.
Induced currents from RF exposure can be measured using a clamp-on induced current meter. Induced currents in the arms and legs can be measured using these devices.
Calibration
No field calibration is available. The CTC arranges for annual calibration.
HRT Availability
Narda 8860
The Narda 8860 personal RF monitor is used to monitor occupational exposures to RF sources within the frequency range of 100 kilohertz (kHz) – 100 gigahertz (GHz). The user can select from multiple alarm settings. The unit continuously logs RF exposure levels and reports results based on a shaped frequency response. Note that the exposure limit for microwave radiation in general industry, see 29 CFR 1910.97(a)(2)(i), is a 6-minute STEL, and that the primary effect of microwave radiation exposure is heating of body tissues, with the most sensitive organs being the eyes and testes.
Appendix A
Instrument Chart
The information shown in the table below is for reference only. Not every field office will have every type of instrument. Refer to the CTC ALEP for specific information, or to the SLTC.
Instrument Use
Physical Measurements |
||
---|---|---|
Type of Instrument |
Measured Substance |
Application |
Stop time meter |
Time |
Calibration |
Tachometers |
Mechanical speed |
Flywheels, belts, cylinders, lathes, etc. |
Ergonomic testing equipment |
Force |
Force measurements for ergonomic assessment |
Electrical testers and multimeters |
Electricity |
Electrical circuits |
Vibration meters |
Vibration |
Handheld power tools, bearings, gear trains, housings, walls |
Thermoanemometer (air velocity meter) |
Airspeed |
Ventilation assessments |
Detector tubes with hand pumps (bellows or piston style) |
Chemical air contaminants |
Screening, spot measurements for air contaminants |
Pressure gauges |
Air Pressure |
Compressor air lines |
Fibrous aerosol monitors |
Fibers in air |
Asbestos |
Dust monitors (particle or respirable aerosol monitors) |
Total dust, respirable dust |
Mines, sandblasting, road construction, dusty operations, indoor air quality |
GAS & VAPOR METERS |
||
---|---|---|
Type of Instrument |
Measured Substance |
Application |
Multi-gas meters |
Combustible gas (LEL), oxygen (O2), with specific toxic gas sensors |
Confined spaces, underground construction, sewers |
Toxic gas sensor, hydrogen sulfide |
H2S |
Farms, sewers, underground construction |
Toxic gas sensor, hydrogen cyanide |
HCN |
Industrial facilities, electroplating operations |
Toxic gas sensor, sulfur dioxide |
SO2 |
Paper mills, bleaching operations |
Toxic gas sensor, nitric oxide and nitrogen dioxide |
NO and NO2 |
Combustion sources, particularly from propane fuel |
Toxic gas sensor, chlorine and chlorine dioxide |
Cl2 and ClO2 |
Bleaching and disinfecting operations, plastics manufacture, chemical synthesis, other industrial operations |
Toxic gas sensor, ammonia |
NH3 |
Industrial refrigeration, fertilizer, animal feed lots |
Toxic gas sensor, phosphine |
PH3 |
Semiconductor manufacture, agricultural pesticides |
Carbon monoxide monitor |
CO |
Garages, warehouses, other combustion sources, indoor air quality |
Carbon dioxide monitor |
CO2 |
Indoor air quality, as a surrogate for other indoor source pollutants |
Infrared analyzers |
CO, CO2, organic substances |
Area surveys to determine locations with highest concentrations, waste anesthetic gases, fumigants, indoor air, leaks, spills |
Photoionization Detectors (PIDs) |
Hydrocarbons, other ionizable substances |
Area surveys to determine locations with highest concentrations, indoor air, leaks, spills |
Mercury vapor meters |
Hg |
Mercury plants, spills |
Ozone Analyzers |
O3 |
Water or air purification, indoor air |
RADIATION METERS |
||
---|---|---|
Type of Instrument |
Measured Substance |
Application |
Heat stress meters |
Ambient (environmental) heat |
Foundries, furnaces, ovens and outdoor work locations |
Light meters |
Light (illumination) |
Indoor lighting, UV exposure |
Microwave meters |
Microwave radiation |
Communications, microwaves, heaters |
Radiofrequency instruments |
Electromagnetic fields |
RF heat sealers, VDTs, induction motors |
Magnetic field testers |
Magnetic Flux Density |
Magnetic fields |
Electrostatic field tester |
Static electric fields |
Hazardous locations |
Ionizing radiation meters |
Ionizing radiation |
Nuclear plants, nuclear waste, laboratory and medical settings |
BIOLOGICAL MONITORS |
||
---|---|---|
Type of Instrument |
Measured Substance |
Application |
Microbial sampler |
Micro-organisms (microbes) |
Indoor air quality |
Appendix B
Chemical Warfare Agent Detection
There are several methods and types of instruments that can be used in the detection of chemical warfare agents, such as nerve, blister, blood, and choking agents. However, most of these agents (nerve and blister) have extremely low occupational exposure limits, and nearly all the detection methods lack the sensitivity required to provide results at these low levels. It is important to understand the capabilities, uses, and limitations of each type of detection device or instrument. The manufacturer of each system provides clear and specific use instructions with each kit. Users should familiarize themselves with these instructions, know the limitations of each device or instrument, and practice the use of the kits while wearing appropriate PPE in a non-contaminated environment. The following sections highlight some types of equipment that are used for detection of chemical warfare agents. Generally, use of these detection systems will be limited to specially trained and equipped personnel at the SLTC or other specially trained and equipped OSHA personnel. The following summarizes specialized direct-reading capabilities that OSHA has access to and should be considered informational by all other personnel. These systems should only be used after consultation with the SLTC and under close SLTC guidance.
A. Military Detection Papers/Kits
-
M8/C8 Detector Paper
The M8 detector paper was developed to detect liquid agents, specifically V- and G-type nerve agents, and H-type blister agents. The C8 paper is equivalent to the M8 paper; the "C" indicates a version manufactured for commercial use. These papers do not detect chemical agent vapors. The sheets are impregnated with chemical compounds that change to green, yellow, or red depending on the type of liquid agent encountered. A color chart accompanying the booklet helps determine the type of agent detected. The result is qualitative, but the detector paper has a sensitivity of about 20 microliters (μL) of liquid. Some substances can act as interferences and produce false positives, such as insecticides, antifreeze, and petroleum products.
A similar product, termed "3-way" paper is also available. This detector paper is equivalent to the M8/C8 papers, except that it includes an adhesive backing that can be used to apply the paper to equipment or PPE.
-
M9 Detector Paper
The M9 paper detects the presence of liquid nerve and blister agents by turning a reddish color. It does not distinguish the type of agent, nor does it detect chemical agent vapors. It will detect a liquid agent droplet with a diameter of approximately 100 micrometers (μm). Interfering substances that will produce a false positive include petroleum products, antifreeze, and insecticides. The papers come in a roll and are adhesive-backed.
-
M256A1 Detector Kit
The M256A1 Chemical Agent Detector Kit is designed to detect and identify chemical agent vapors, including blood (AC and CK), blister (H, HN, HD, CX, L), and nerve (V and G series) agents. The test consists of a series of chemical ampoules that are broken and exposed to the air. The reagents in the ampoules react with chemical agent vapors to produce a color change. A color chart and instructions included with the kit are used to determine the type of agent(s) that is/are present. The M256A1 is relatively sensitive, and can detect some of the agents below the IDLH levels. The kit also includes booklets of M8 paper for detecting liquid agents.
-
C-2 Detector Kit
The C-2 Chemical Agent Detector Kit is used by the Canadian Military for detecting chemical agent vapors. The C-2 kit utilizes various colorimetric detection tubes for identifying nerve, blister, blood, and choking agents. Similar to the M256A1 kit, it will allow detection of some agents below IDLH levels. It also contains a booklet of M8 paper for use with detection of liquid agents.
B. Colorimetric Tubes
Colorimetric tubes are made by several manufacturers, and their function is essentially the same. They contain a series of tubes which can be used to detect airborne chemical agents, as well as toxic industrial chemicals. Conducting a single test with one or more tubes takes two to five minutes to complete. There are some tubes, such as those for blister and nerve agents, which give a qualitative detection of the presence of that family of chemicals up to near IDLH levels. The industrial agents (blood agents and choking agents) can be specifically identified and quantitatively measured in ppm to levels below applicable exposure limits. An example of colorimetric tubes designed specifically for chemical agents is the Dräger Civil Defense Simultest™ (CDS) Kit.
C. Portable Chemical Agent Detectors
Most types of portable, traditional chemical detection equipment, such as photoionization detectors, flame ionization detectors, electrochemical sensors, infrared analyzers, etc. can be used for chemical agent detection. These types of instruments are discussed in other sections of the Technical Manual. However, due to the acute toxicity of chemical warfare agents at very low concentrations, these instruments lack adequate sensitivity and cannot provide detection below IDLH levels. Some instruments have been developed for use specifically with chemical agents, and research is ongoing. Some of the more popular technologies and instruments are discussed below.
-
Ion Mobility Spectrometers
An ion mobility spectrometer (IMS) operates by drawing air into the instrument where it is ionized with a radioactive source. The ionized molecules travel through a charged tube, where they become separated according to their mass and mobility before reaching a collector electrode. An electronic signature is produced for each ion, which gives an indication of the type and relative concentration of agent present. IMS detectors are used mainly to detect nerve, blister, and blood agents. Examples of IMS detectors include the Chemical Agent Monitor (CAM), Improved Chemical Agent Monitor (ICAM), APD 2000 (Advanced Portable Detector), and SABRE 4000.
These instruments will not detect at levels below IDLH for most chemical agents. They are best used for site reconnaissance, or to screen for contamination on equipment or personnel. Some interferents that may cause false alarms with an IMS include the following: cleaning compounds and disinfectants that contain additives such as menthol and methyl salicylate (oil of wintergreen); aromatic vapors, such as perfumes and food flavorings; and exhaust from some motors and fumes from explosives and propellants.
-
Surface Acoustic Wave
Surface acoustic wave (SAW) sensors are comprised of piezoelectric crystals with selective surface coatings. As the mass of a chemical vapor sample flows over the sensors, it is absorbed onto the surface which results in a change in vibration frequency of the sensor. An internal microprocessor in the instrument measures these changes, providing detection and identification of the chemical agent. Portable instruments utilizing SAW technology are available for detection of nerve and blister agents. Examples of SAW instruments include the HAZMATCAD and SAW MiniCAD. As with IMS detectors, SAW instruments will not allow detection of most chemical agents below IDLH levels. However, SAW detectors are less susceptible to false positive alarms from interfering substances.
D. Gas Chromatographs/Mass Spectrometers
Additional instruments that can be used for chemical agent detection and identification are gas chromatographs (GC) and mass spectrometers (MS). These are generally laboratory-type instruments which require skilled laboratory technicians for operation and interpretation of results. A few have been hardened for use in vans and portable handheld units can be used in the field; however, the technicians normally must collect a sample from the suspect material and bring it to the instrument. Currently, the GC or GC/MS is the only instrument that can verify the concentrations of nerve agents down to levels which are below applicable TWA occupational exposure limits. TWA levels (PEL/TLV). This is important for applications where it is important in determining the appropriate types and levels of PPE or to verify that decontamination is complete.
An example of a portable GC is the MINICAMS Continuous Air Monitor. This instrument is used extensively in Department of Defense depots where chemical agents are stored and used by other agencies in the field. The MINICAMS can provide automatic, quantitative identification of the chemical agents for which it was calibrated.
E. HRT Availability
The following equipment is maintained by the HRT for use in chemical agent detection:
- Military Detection Papers/Kits: M8/C8 paper, M9 paper, M256A1 kits, and C-2 kits.
- APD 2000; 2 IMS detection units as described above. Each OSHA region is also equipped with a single APD 2000 unit.
NOTE: The HRT also serves as the coordinator for OSHA's SRTs and can provide additional assistance and technical information regarding chemical warfare agent detection. Special precautions, such as PPE and/or other work practices, are also necessary to prevent exposure when working with chemical warfare agents. Contact the HRT for more details.
Appendix C
Biological Agent Detection
Sampling and analysis for biological agents is a rapidly growing field. Many techniques and technologies are still under development. There are various factors to consider when sampling for biological agents, such as: method of dispersion for the agent, purpose of the sampling (e.g., to identify the agent, determine extent of contamination, confirm decontamination, etc.), environmental conditions, persistence of the agent, physical state of the agent, area/volume to be sampled, laboratory protocols, and others. It is important to note that biological agents (such as bacteria, viruses, and endotoxins) are particulate matter, and, therefore, detection methods are designed for particulate sampling. The following sections highlight some types of equipment that may be used for sampling and detection of biological agents.
A. Surface/Bulk Sampling
-
Swabs
Swabs have been used frequently when sampling surface areas for the presence of biological agents. Swab tips come in a variety of materials, such as cotton, Dacron™, polyester, rayon, and foam. Shafts can be comprised of either wood or plastic. Generally, synthetic swab tips with plastic shafts are recommended because they are not of biological origin and will not interfere with DNA-based detection systems. Swabs may be used dry or wetted with a buffer solution. In general, studies have shown that wet swabs have higher collection efficiency than dry swabs.
-
Wipes and Sponges
Wipes and sponges are often used because they can sample larger surface areas and have a higher collection efficiency compared to swabs. They can also be used in a dry or wet fashion. Various styles and materials for wipes and sponges are available. As with swabs, synthetic materials are recommended to eliminate potential interference problems with detection systems.
-
Vacuum Methods
Vacuum methods can be used when it is necessary to sample very large surface areas or surfaces which are porous or irregular such as carpeting, where it is impractical to use swabs or wipes. These methods are also useful to gather bulk dust samples for analysis. One method utilizes a HEPA-filtered vacuum equipped with a dust collection filter sock which is used to capture the sample. Large surface areas can be vacuumed, and the dust gathered in the sock is then analyzed for the presence of biological agents. A similar method uses a portable sampling pump equipped with a filter cassette to "vacuum" particulate matter from smaller areas, and at lower flow rates. The filter can then be analyzed for biological agents.
-
Agar Plates
Agar plates, also known as "sticky plates," can be used to sample a surface by contacting the plate directly to the surface. The particles from the surface will adhere to the plate, which can then be analyzed by culture to identify any biological agents. This method has been used by various agencies during investigations of incidents involving biological agents.
B. Air Sampling
Air sampling can be performed to determine the presence of airborne biological particulates. Essentially, a volume of air is drawn through a filter or deposited in another medium, and the captured particulates are then analyzed to identify biological agents. High flow rates are generally desirable because this allows higher sample volumes and increases the likelihood of detecting the suspect agents. However, it should be noted that some organisms are fragile, and the high velocities and impact mechanisms may kill the organism during the sampling process. Consult with SLTC to determine the appropriate flow rate and procedures for your situation.
Low flow air sampling methods consist of traditional personal sampling pumps equipped with capture devices such as filter media or liquid impingers. These low flow methods have the advantage of being small and portable; however, due to their low sample volume they will have a relatively high limit of detection.
Impactors, such as the Six Stage Viable Andersen Cascade Impactor, utilize higher flow rates (around 30 L/min), sample a greater air volume, and, therefore, increase the likelihood of detecting the agent. This and similar types of impactors capture the biological particulate directly on an agar plate which can then be analyzed in a laboratory by culture method.
High volume area samplers are also available for biological agents. These samplers possess flow rates ranging from 200 to 600 L/min, so they are able to sample very large volumes of air. Some instruments deposit the particulate matter on a filter, while others capture it in a liquid solution.
C. Generic Detection
There are several techniques and instruments available that will allow responders to perform a generic detection for biological agents. These methods will not identify a specific agent, but can be used to determine if a suspect material is of biological origin, and to rule out hoax materials. The following are some examples of equipment types:
- Particle Analyzers: The particle size of a sample can be analyzed and compared to known size ranges for biological materials. If the particle size is too large or too small, biological materials can be ruled out.
- Fluorometer: These instruments will detect the presence of DNA, which is a component of most biological materials. A positive response by the meter for a given sample indicates a biological material, but again, does not identify the material or agent.
- Luminometer: A luminometer operates similarly to a fluorometer, except that it will detect the presence of adenosine triphosphate (ATP) in a sample. ATP is another component of a cellular organism, thereby indicating a biological material.
- Colorimeter: Colorimeters can be used to detect protein from a sample. Again, protein is present in biological organisms, so these instruments can indicate if the material is biological in origin.
- Protein Paper: Similar to a colorimeter, these paper strips can indicate if a given sample contains protein, and is, therefore, biological.
- pH Paper: The pH of a sample is tested with pH paper strips; if the pH range is between 5 and 9, the material may be biological. If the pH is outside this range (below 5 or above 9), then biological materials can be ruled out.
D. Identification
-
Immunoassay/Handheld Assay
An immunoassay test, also known as a handheld assay (HHA), can be performed on a sample to identify a specific agent. These HHA tests rely on an antigen/antibody reaction to identify the suspect agent. The test is presumptive, meaning that a given agent must be suspected and then tested with its specific HHA for confirmation. For example, if Bacillus anthracis (anthrax) is suspected, the sample is tested using an HHA designed for Bacillus anthracis; a positive result confirms the presence of the organism while a negative result indicates that the sample does not contain that specific organism. The HHA units are small, the test can be performed in the field, and they rely on a visual colorimetric change for sample results. Some HHA systems come with an electronic reader to aid in detecting the colorimetric change.
HHAs are under scrutiny due to limitations on sensitivity and specificity; i.e., high rates of false-negative and false-positive results. The results from an HHA test should not be relied upon alone and further confirmatory analysis should always be performed. However, these tests are used widely by first responders as a rapid field test. Although they are presumptive, their results can assist decision makers in taking protective actions, treating potential infections, and involving other authorities as necessary.
-
Polymerase Chain Reaction
Polymerase chain reaction (PCR) is a system that allows identification of an agent based on its DNA. The DNA from the sample is obtained and reproduced rapidly to produce a quantity that is detectable by the instrumentation. For example, after 30 cycles with the PCR system, one copy of DNA from an agent sample can be reproduced until there are one billion copies, which can then be analyzed and identified.
PCR is performed real-time through detection by fluorescence. During the PCR cycle, DNA-specific "probes" with fluorescent dyes are attached to the DNA sample which allows detection. PCR can be performed in a laboratory, or in the field with semi-portable instrumentation. Specific reagents and supplies are necessary to perform the analysis.
PCR has been useful for biological agent detection because it has excellent sensitivity, good specificity, and provides real-time results. Some weaknesses of PCR to consider are the following: potential interferences from other substances in the sample, reagent stability, and sample viability, because PCR will detect the presence of both live and dead organisms, but will not distinguish between the two.
-
Culture
Analysis by culture is considered by many to be the "gold standard" for the identification of biological agents. Samples are sent to a laboratory where they are prepared and applied to an agar plate on which the suspect biological organisms are allowed to grow. After a sufficient period of time (usually 24 hours or more), visible growth can be examined to detect the presence of the biological agent(s). Often, culture is used for the confirmatory analysis of previous detection methods for a given sample (HHAs, PCR). Some disadvantages of culture include delayed results and the procedure will only detect living organisms. Any biological agent that has died before the analysis has begun will not be detected. Note that biological toxins or allergens associated with nonviable/nonculturable agents may still cause health effects.
HRT Availability
The following equipment is maintained by the HRT for use in biological agent sampling and analysis:
- Handheld Assays for the following agents: anthrax, plague, brucellosis, tularemia, Venezuelan equine encephalitis, staphylococcal enterotoxin B, botulinum toxin, ricin, smallpox, and Q fever.
- HEPA Vacuums: Two units with filter socks and other supplies specifically for use with biological agent sampling.
- Andersen Cascade Impactors: Two units.
- Dry Filter Units (DFU): Two units. The DFU is a high-volume air sampler designed for biological agent sampling. It operates at flow rates up to 600 L/min and utilizes a filter pad for capturing the agent.
- Surface/Bulk Sampling: Various wipe, sponge, and swab sampling kits.
NOTE: The HRT also serves as the coordinator for OSHA's biological SRT and can provide additional assistance and technical information regarding biological agent detection. Special precautions such as PPE and/or other work practices are also necessary to prevent exposure when working with biological agents. This information is provided as a reference for specially trained personnel and is not generally intended for CSHO use. Contact the HRT for more details.
Appendix D
Ionizing Radiation Monitors and Meters
The following sections contain a brief description of the types of instruments that may be used for monitoring exposures to ionizing radiation and radioactive materials.
A. Survey Meters
Application and Principle of Operation
Radiation survey meters are used to locate and quantify sources of ionizing radiation or to quantify the exposure rate from sources of ionizing radiation. To assess the quantity of radioactive materials present, survey meters are typically calibrated to measure counts per minute (cpm). To measure the exposure rate from gamma (γ) or X radiation sources, survey meters are calibrated to measure roentgens per hours (R/h). Most survey meters have either gas filled detectors or scintillation detectors. Not all survey meters are configured to measure all radiation types. Survey meters must be chosen based on the type and energy of the radiation you expect to measure and whether you wish to measure cpm or R/h.
Calibration
Calibration is performed by the manufacturer on a periodic (usually annual) basis.
HRT Availability
-
Ludlum Model 3 with 44-9 Pancake GM Detector
The Ludlum Model 3 is a general purpose survey meter fitted with a pancake Geiger Mueller (GM) detector capable of measuring alpha (α), beta (β), γ, and X radiations. This instrument will measure count rates over a range of 0–500,000 cpm.
-
Ludlum Model 2360 with 43-93 Alpha/Beta Scintillator
The Ludlum Model 2360 is a survey meter capable of measuring and discriminating between α and β radiations. It is fitted with a Ludlum 43-93 alpha/beta scintillation detector. The meter will measure count rates over a range of 0–500,000 cpm.
-
Ludlum Model 192 MicroR™ Meter
The Ludlum Model 192 is a low level (μR/h) γ and X radiation exposure rate meter. The meter has an internal sodium iodide detector capable of measuring dose rates between 0 and 5,000 μR/h.
-
Thermo Scientific® FH40GL Dose Rate Meter with FHZ732GM Pancake Probe
The FH40GL is a stand-alone radiation survey meter equipped with an internal proportional detector capable of measuring γ and X radiation exposure rates from 1 μR/h–10 R/h. The unit is also equipped with an FHX732GM pancake GM detector capable of measuring count rates from α, β, and γ radiations over a range of 0.01–100,000 counts per second.
-
Thermo Scientific® FH40TG Teleprobe™
The FH40TG telescoping probe can be used with the FH40GL to measure γ and X radiation exposure rates over a range of 10 μR/h–1,000 R/h. The probe is equipped with two GM detectors that can be extended up to 13 feet away from the user, allowing exposure rate measurements to be made at a distance from the source.
-
Thermo Scientific® RO-20 Ionization Chamber
The RO-20 is capable of measuring exposure rates from β, γ, and X radiations. The instrument is equipped with an air filled ionization detector capable of measuring exposure rates up to 50 R/h.
-
Thermo Scientific® PM1703M Gamma Pager
The PM1703M is a pager-sized survey meter that can be worn on the belt. The meter contains a cesium iodide scintillator-photodiode detector capable of measuring γ and X radiation. The instrument will measure exposure rates from 0–5,000 μR/h. The PM1703M is a highly sensitive instrument that can be set to alarm when the background varies by a user-set factor. This meter can be used to warn the user that he/she has entered a radiation area that is above background radiation levels.
B. Scalars
Application and Principle of Operation
Scalars are used to analyze samples of radioactive material and to quantify the amount of material present. They are often used to measure the amount of radioactive material in air samples, wipe samples, and nasal swabs. Scalars use the same detector types used in survey meters. These instruments can typically be set to count a sample for a specified time.
Calibration
Calibration is performed by the manufacturer on a periodic (usually annual) basis.
HRT Availability
-
Ludlum Model 3030 Alpha/Beta Scalar
The Ludlum Model 3030 is a dual alpha/beta scalar used for sample counting. The instrument has a silver-activated zinc sulfide [ZnS(Ag)] coated scintillation detector capable of discriminating between α and β radiations. The readout on the front of the instrument reports both α and β counts for the specified period. Counting time can be set from 0.1–30 minutes.
-
Ludlum Model 2000 Scalar with 43-10 Alpha Sample Counter
The Ludlum Model 2000 scalar with 43-10 sample counter is capable of counting samples for α particle emissions. The sample counter has a ZnS(Ag) scintillation detector. Counting time can be set from 6–990 minutes.
-
Thermo HandECount Scalar
The HandECount is a battery or AC powered sample counter used for determining the α and β activity present in a sample. The instrument is controlled by a Palm™ hand-held computer platform and operating system which communicates with a standard modular detector board to perform all counting operations. All data is automatically logged to a file for later retrieval to a PC. The HandECount will report both α and β counts for a sample.
C. Electronic Personal Dosimeters
Application and Principle of Operation
Electronic personal dosimeters are used to measure the dose received by an individual. They are normally worn on the front of the body in the chest area. Most electronic dosimeters measure the deep dose equivalent (Hp(10)) to γ radiation. Some electronic dosimeters also measure the shallow dose equivalent (Hp(0.07)). Most electronic dosimeters allow the user to set alarms for integrated dose and/or dose rates.
Calibration
Calibration is performed by the manufacturer on a periodic (usually annual) basis.
HRT Availability
-
Thermo Electronic Personal Dosimeter (EPD) Mk.2
The Thermo EPD Mk.2 is an electronic dosimeter capable of measuring Hp(10) (deep dose) and Hp(0.07) (shallow/skin dose). It is sensitive to γ and X radiations for Hp(10) measurements, and is sensitive to γ, β, and X radiations for Hp(0.07) measurements. Alarms can be set for accumulated doses and for dose rates for both Hp(10) and Hp(0.07).
-
Rados RAD-60 Electronic Personal Dosimeters
The Rados RAD-60 is capable of measuring Hp(10) from γ and X radiations. Alarms can be set for both dose and dose rate.
D. Spectroscopy
Application and Principle of Operation
Portable handheld radiation spectroscopy instruments allow the user to identify radionuclides. These instruments typically use a sodium iodide detector with a multichannel analyzer to measure the energy spectrum emitted by a radioactive source. The instrument compares the spectrum to a library of spectra and provides the user with a list of likely sources. Spectra can also be downloaded to a computer if the user wishes to perform the spectral analysis manually or wishes to print the spectra for documentation.
Calibration
Calibration is performed by the manufacturer on a periodic (usually annual) basis.
HRT Availability
EXPLORANIUM™ GR-135N
The EXPLORANIUM™ GR-135N is a handheld isotope identification device. The GR-135N has a sodium iodide detector capable of identifying radionuclides, a GM detector for measuring exposure rate, and a solid state neutron detector. Spectra from the GR-135N can be downloaded to a computer for analysis and printing.
E. Electret-Passive Environmental Radon Monitoring
Application and Principle of Operation
The Electret-Passive Environmental Radon Monitor (E-PERM) system is a passive integrating detector system for the measurement of radon (222Rn) or thoron (220Rn) concentrations in air. It consists of a charged Teflon® disk (electret), an open-faced ionization chamber, and an electret voltage reader. When the electret is screwed into the chamber, an electrostatic field is established and a passive ionization chamber is formed. The chamber is deployed directly in the area to be measured. Radon gas diffuses passively into the chamber and the α particles emitted from the decay of radon ionize the air molecules. These ions are then attracted to the charged surface of the electret, and the charge on the electret is reduced. The electret charge is measured before and after the exposure with a portable electret voltage reader, and the rate of change of the charge (change divided by the time of exposure) is proportional to the concentration of radon in the area.
Calibration
Calibration factors are provided for each type of electret. Calibration factors are voltage dependant and instructions for calculating the calibration factors are in the E-PERM manual provided by the manufacturer.
HRT Availability
The HRT has 12 E-PERM chambers, electrets, and a voltage reader.
F. Radiation PPE Shielding
In radioactively contaminated areas, PPE is typically used in order to prevent workers from becoming contaminated, and to minimize the spread of radioactive contamination. The choice of appropriate shielding for ionizing radiation depends on the type and energy of the radiations to be shielded. Alpha particles have very low penetrating power and travel only a few centimeters in air and will not penetrate the dead outer layer of skin. Shielding is generally not required for alpha particles because external exposure to alpha particles delivers no dose. Where particulates contaminated with alpha particles are present, HEPA-filtered respiratory protection is critical to prevent an internal dose. Beta particles can travel several meters in air and can penetrate several millimeters into the skin. Beta particles should be shielded using an appropriate thickness of low atomic mass (low-Z) materials such as aluminum or plastics (e.g., Plexiglas®). Shielding beta particles with high-Z materials should be avoided as this can result in production of secondary X radiation (i.e., bremsstrahlung radiation). Gamma and X-rays can travel kilometers in air and can penetrate deep into the human body or pass through it entirely. Gamma and X-rays are most efficiently shielded using an appropriate thickness of high-Z materials such as lead or steel, or with an appropriate thickness of concrete. Neutrons are most efficiently shielded using an appropriate thickness of hydrogenous materials such as paraffin, water, or plastics, or with an appropriate thickness of concrete.
NOTE: The HRT also serves as the coordinator for OSHA's radiation SRT and can provide additional assistance and technical information regarding radiation measurements. Special precautions are also necessary to prevent exposure when working with radioactive materials, such as PPE and/or other work practices. Contact the HRT for more details.